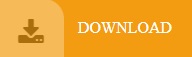
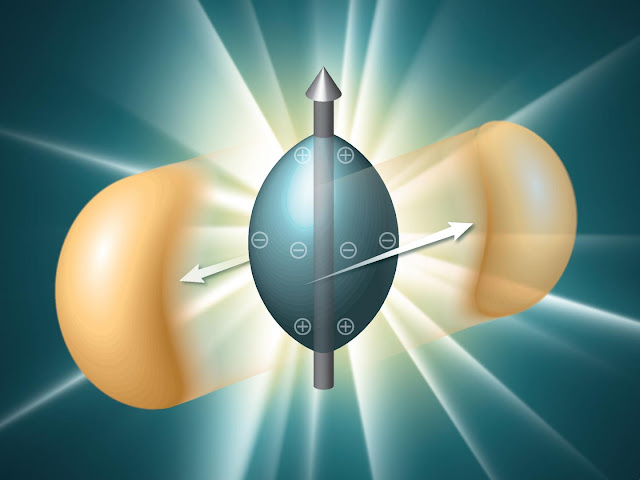
By repurposing the waste and incorporating it into PEO coatings, we can reduce the amount of waste generated by the industry and create a more sustainable future. This is where the concept of recycling particles from photovoltaics into PEO coatings comes in. While these solutions are a step in the right direction, there is still much work to be done to address the growing problem of photovoltaic waste. There are several current solutions to photovoltaic waste, including recycling and repurposing. According to a report by the International Renewable Energy Agency (IRENA), the amount of solar panel waste could reach 78 million metric tons by 2050. These materials are used to manufacture solar panels, and they can have a significant impact on the environment if not properly disposed of. Photovoltaic waste is made up of various materials, including glass, silicon, and rare metals such as cadmium, tellurium, and indium. We will also discuss the benefits of PEO coatings and the challenges of incorporating photovoltaic waste. In this article, we will discuss photovoltaic wastes and PEO coatings and explore the potential of PEO coatings to reduce the impact of photovoltaic waste. However, there may be a solution in the form of plasma electrolytic oxidation (PEO) coatings.īy recycling particles from photovoltaics into PEO coatings, we can reuse waste and create a more sustainable future. This waste contains harmful materials that can harm the environment. As photovoltaic technology gains popularity, so does the amount of waste it generates.
#Plasma particles full#
Any reuse without express permission from the copyright owner is prohibited.The clean energy revolution is in full swing, but with it comes a new problem: waste. Published on 16 December 2020.Įxcept where otherwise noted, images are subject to copyright. (2020), A new approach to characterizing space plasmas, Eos, 101. ( Journal of Geophysical Research: Space Physics,, 2020)

Nevertheless, the study reinforces the need for care when interpreting complicated particle distributions. The authors suggest that a multibeam approach offers clear advantages when interpreting energy transport in complex plasmas, although they note that the approach is based on assumptions, such as the number of beams into which a given distribution should be decomposed. Using the new approach yields the more intuitive result that the multibeam system has all kinetic energy and no thermal energy. This approach enables better understanding of how much of the system’s overall fluid energy density is kinetic (i.e., associated with the beam flow velocities) and how much is thermal (i.e., associated with velocity fluctuations about the beam flow velocities).

address such misinterpretations by developing methods for taking a new kind of multibeam velocity moment of a measured distribution, in which multiple beams are identified and their single-fluid thermal moments are added together. Because their velocities cancel out when averaged, single-fluid moments can appear to contain all thermal energy and no bulk kinetic energy. Imagine, for example, two equal beams of particles moving in opposite directions with all particles moving at the same speed. Taking single-fluid moments of such multibeam distributions can yield counterintuitive results. These high-resolution distributions frequently show more than one distinct velocity peak, or beam, present at the same time in the same spatial region. The satellites in NASA’s Magnetospheric Multiscale Mission have recently measured kinetic particle distributions over time intervals much faster than in earlier missions.

Second-order moments yield thermal and kinetic energy densities, which describe the single-fluid temperature and bulk kinetic energy associated with the flow velocity. Zero- and first-order moments yield the plasma’s fluid density and particle flux and hence the single-fluid flow velocity.
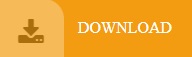